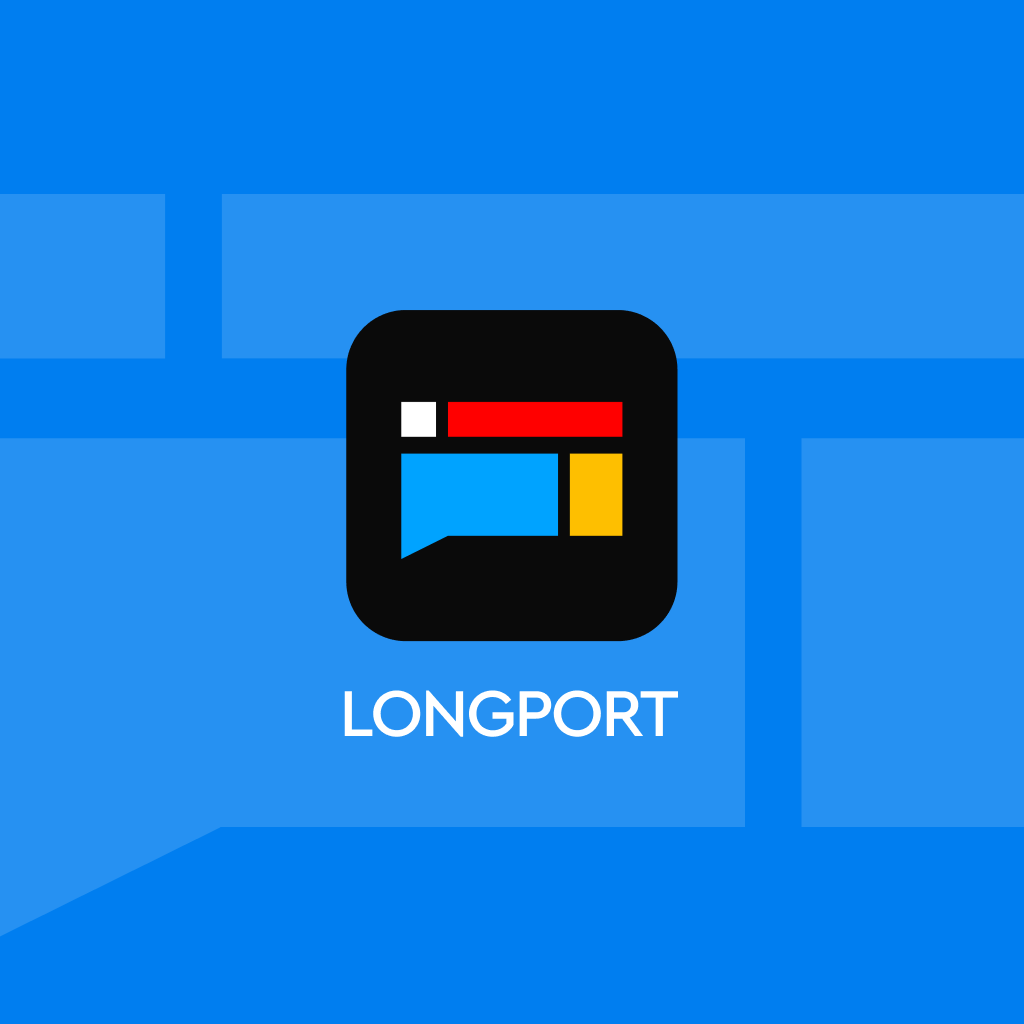
Popular Science | The Past, Present, and Future of Chips
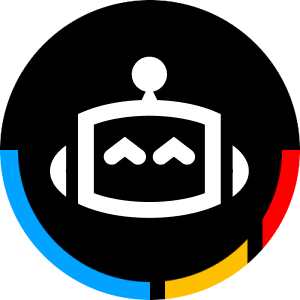
A brief introduction to chips.
This article is a compilation from Yuan Strategy and the internet.
Chips constitute the core control unit for the normal operation of modern society, with its influence spanning national security, technology, politics, culture, and more. Without chips, everything we have now would be impossible to discuss.
As the cornerstone of intelligent, computing, and electronic industries, the advancement of chips determines the height of future technological development. To grasp our fate in the AI revolution, we need to review the history of chips and look ahead to the future.
01 What is a Chip?
A chip, also known as an Integrated Circuit (IC), or microcircuit, microchip, or simply chip, is a small yet powerful electronic component containing billions of tiny electronic elements like transistors and capacitors. These elements are interconnected to perform various computing and storage tasks. Chips are the core of modern electronic devices, whether it's smartphones, computers, cars, or home appliances, all rely on chips to function.
02 Moore's Law, the Inescapable Rule for Chips
(Moore's Law)
In the modern era of technology, Moore's Law is considered a crucial and omnipresent rule. This law was proposed by Gordon Moore, one of the founders of Intel, in 1965. Its core idea can be succinctly summarized as: the number of transistors that can be accommodated on an integrated circuit will double approximately every 18 months to 2 years, while the price becomes increasingly cheaper. Although named after Moore, this law actually represents the development direction of the entire computer field. Moore's Law is so important because it has had a profound impact on our lives.
Firstly, it drives continuous advancement in computers. Remember smartphones from a decade ago? Their performance may not match that of a mid-range phone today. Moore's Law makes our devices faster and more powerful, giving rise to countless applications and services, from smart assistants to virtual reality.
Secondly, Moore's Law propels the vigorous development of the information technology industry. The chip manufacturing industry has become one of the world's largest industries, bringing significant economic growth and creating numerous job opportunities. It also accelerates scientific research progress, as enhanced computing power can be used for simulating, analyzing, and solving complex scientific problems.
However, like all things, Moore's Law also faces challenges. As transistors continue to shrink, we are approaching the physical limits of silicon-based chips, leading to increased manufacturing costs, heat issues, and problems like battery life. To continue following this law, scientists are actively seeking new technological breakthroughs, such as quantum computing and photon computing.
The Three Major Types of Chips
Currently, chips are mainly divided into three major types:
The first type is logic chips, which refers to processors running smartphones, computers, and servers.
Logic chips are an indispensable key component in modern electronic devices. There are various types of logic chips, including Central Processing Units (CPUs), Graphics Processing Units (GPUs), Field-Programmable Gate Arrays (FPGAs), etc. These compact chips have excellent logical processing capabilities, and their core task is to execute various decision-making and control tasks, just like the brain of electronic devices.
Whether it's household appliances, automotive control systems, or industrial automation, logic chips quietly play a role behind the scenes to ensure the normal operation of equipment. These compact "brains" can quickly process massive amounts of data, make complex decisions, and make our lives more convenient, safe, and efficient.
(Flash memory chip)
The second type is storage chips, which refers to NAND Flash chips and Dynamic Random-Access Memory (DRAM). Storage chips are widely used in modern tech devices and are one of the cornerstones of the digital information age. These powerful chips act as warehouses for data, storing our valuable information in electronic devices, ensuring long-term storage and instant access to data.
One of the most common types of storage chips is flash memory chips, which are found in various devices such as USB drives, phones, cameras, etc. These chips are used to store information, with features of high speed, large capacity, and durability, allowing us to store and transfer photos, files, and videos anytime, anywhere.
Another common type of storage chip is Dynamic Random-Access Memory (DRAM), which is used for temporary data storage. Its speed and storage capacity enable our computing devices to process information at unprecedented speeds. These DRAMs are present in our computers, smartphones, tablets, and many other devices, serving as temporary memory. When you open applications, browse the web, or edit documents, DRAM quickly stores and provides the required data for the CPU to efficiently perform calculations and operations. This high-speed data read-write capability is crucial for modern computing as it ensures that your device can respond to your commands in real-time.
Storage chips not only play a role in personal devices but also play a crucial role in large-scale data centers and cloud computing. The continuous development of these chips enables us to store and process vast amounts of data, driving advancements in fields such as scientific research, business analytics, and entertainment media.
Simulated Chips
The third category of chips is relatively diverse, including analog chips such as sensors that convert visual or audio signals into digital data, RF chips that communicate with mobile networks, and chips that manage how devices use power.
Analog chips are microelectronic chips with powerful analog signal processing capabilities, focusing on processing continuous signals such as sound, light, voltage, etc., enabling our electronic devices to better simulate and interact with the real world. Analog chips are widely used in various fields. In audio devices, they help us capture and process sound, making music, phone calls, and voice recognition possible; in the medical field, they are used to monitor physiological signals such as electrocardiograms and blood pressure, helping doctors make accurate diagnoses; in the communication field, analog chips are used for wireless communication, radar, and satellite communication, ensuring efficient information transmission.
RF chips are key components in the wireless communication world. They can send, receive, and process RF signals, allowing our mobile phones, Wi-Fi routers, satellite navigation, TVs, and other devices to communicate wirelessly. RF chips can transmit data in different frequency bands, from short-range Bluetooth connections to long-distance satellite communication, covering all aspects. Their presence allows us to connect with information and people from around the world anytime, anywhere. Regardless of where you use wireless technology, RF chips play an indispensable role, providing us with convenient and efficient wireless communication.
The third category does not primarily rely on Moore's Law to drive performance improvements; smart design is more important than shrinking transistors. Manufacturers of these chips typically do not need to compete to make smaller transistors every few years, so their costs are much lower, averaging only a quarter of the capital investment of advanced logic chip or memory chip manufacturers.
Timeline of Chip Development
(Source: "A Brief History of Chips")
Chip Development Tree
(From basic disciplines to semiconductor transistors, and then to various chips and applications) Source: "A Brief History of Chips"
The history of chip development is like an epic saga, originating from the exploration of basic disciplines, evolving to the pinnacle of innovation today. It begins with quantum physics, exploring the mysteries of the micro world, revealing the behavior laws of electrons for us. The development of semiconductor physics applies the behavior of electrons to practical use, giving birth to transistors, like a door opening, leading to the path of the digital era.
Since then, the evolution of chips has been like an electronic race, constantly innovating.
1. Quantum Physics
The development of chips originated from the rise of quantum physics, a discipline born in the early 20th century with a history of over 120 years. Quantum physics provided a solid foundation for later chip technology by helping scientists delve into the movement of electrons inside atoms. A profound understanding of quantum physics enables us to manufacture small yet powerful chips, silently supporting the vigorous development of modern technology and allowing us to enjoy a more convenient and efficient way of life.
2. Semiconductor Physics
Semiconductors are materials that can strike a balance between conductors (such as copper) and insulators (such as plastic), with their electrical conductivity falling between the two. This characteristic makes semiconductors an ideal material for chip manufacturing. By controlling the behavior of electrons in semiconductors, scientists can create tiny electronic components like transistors, which are the basic building blocks of chips. The switch function of transistors allows the flow or stoppage of electric current, forming the foundation for computing and information storage. Therefore, semiconductor physics provides the theoretical and material basis for chip technology, driving the development of modern electronic devices.
3. Transistors
As early as 1947, William Shockley and others at Bell Labs in the United States invented transistors, considered a milestone in the electronics industry. Transistors are tiny electronic switches that control the flow or stoppage of electric current, serving as the fundamental components of modern chips. Thousands of transistors on a chip work together to perform various computing and storage tasks. They process information at lightning speed, making our electronic devices fast and efficient.
06 The Future of Chips
1. Cutting-edge Technology I: New Principle Devices
What are new principle devices? The transistor technology of silicon semiconductors is nearing its limit, and we cannot expect too much in terms of further miniaturization.
Currently, there is hope in materials like carbon nanotubes or graphene, which are low-dimensional materials. Their biggest advantage is that the speed of electron transmission in them is much faster than in silicon materials. The purpose of continuously reducing the size of transistors is to make devices faster and more energy-efficient. The only way to achieve this is by making the transistor channels shorter, so that the transmission of information takes less time.
However, when we switch to materials like carbon nanotubes or graphene, the game changes. These low-dimensional materials, even if the devices are not made as small as 5 nanometers or 3 nanometers, could potentially achieve the same processing time and low power consumption. This opens up greater potential for device performance improvement.
In addition to these new low-dimensional materials, there is another emerging device principle worth noting, which is spintronics.
Spin is a natural physical dimension of electrons, essential for quantum computing. In fact, spin also has significant applications in classical computing.
In simple terms, spin refers to the clockwise or counterclockwise rotation of electrons, which can represent 0 and 1. Therefore, with spintronic devices, the switch does not require electrons to flow between two "stations", but simply relies on the flipping of electron spins.
This eliminates the transmission time and power consumption caused by electron flow, making spintronic devices promising for achieving high-speed and low-power operation.
However, the fabrication challenges of these new materials and structures are immense, especially when aiming to produce integrated circuits on the scale of hundreds of millions or even billions of devices, while ensuring uniformity and reliability.
These technologies are currently only in the small-scale research phase in laboratories, but they hold great potential for future development.
2. Cutting-edge Technology II: Reconfigurable Chips
Traditional CPU or GPU chips are fixed in hardware but highly programmable in software, making them general-purpose chips.
Custom chips like Google's TPU have fixed hardware, offering high performance in exchange for flexibility. While FPGAs can be programmed in hardware, it is a static form of programmability that cannot make real-time adjustments based on software.
The term "reconfigurable" means that the internal circuit structure of the chip can be dynamically adjusted according to software, aiming to achieve customized hardware performance for different software applications.
The working principle can be likened to switch points in a railway network, where railway workers switch the tracks to change the train routes. In reconfigurable chips, there are numerous switch points, with each one being a transistor switch that can be rapidly adjusted. This enables real-time adjustments of the circuit structure based on specific problems, achieving a high level of hardware-software integration.
Why develop reconfigurable chips? Specialized chips have been a key driver of the recent AI boom. In the future, to meet the demands of the Internet of Things, we will need more varieties of small-batch specialized chips. However, the dilemma with specialized chips is their high cost, which only large companies like Google and Alibaba can afford.
Therefore, considering that general-purpose chips like CPUs and GPUs can run various software applications, being compatible with Lenovo and Apple computers, capable of running Windows programs as well as Linux and macOS, the high R&D costs of chip development, reaching billions of dollars, can be reasonably distributed across each chip. This kind of positive feedback in the economy has promoted the development of chip technology. However, the high cost of specialized chips is not supported by such a large sales volume, naturally creating a contradiction between performance and economy.
Naturally, we hope for high-performance and cost-effective chips. For example, a chip that can adapt to various types of problems and applications to form an optimal architecture. In other words, this chip is a customized chip for any problem and can efficiently solve problems.
Reconfigurable chip technology aims to achieve this goal. For new problems, when the software changes, the hardware can adapt to the software requirements in a matter of tens of nanoseconds.
Because a single chip can address a large number of different scenarios, there is enough sales volume to spread the development costs. Reconfigurable chip technology is one of the most cutting-edge fields today, and in the next decade, there will definitely be significant breakthroughs in this area.
3. Cutting-edge Technology III: Hybrid of Classical Chips and Quantum Computing
A brand-new chip architecture with great potential for the future.
One significant possibility is the combination of classical chips and quantum computing into a hybrid computing chip group. Once achieved, it will undoubtedly bring a significant improvement to the information industry.
Quantum computing is not meant to replace current classical computers but to complement and work together with them.
One major application scenario of quantum computing is for large-scale optimization problems, achieving exponential acceleration far beyond classical computing systems. However, quantum computing is still far from mature and is believed to remain in the NISQ stage for a long time, which is the stage of noisy intermediate-scale quantum computing, unable to solve large-scale practical problems as ideally envisioned.
Therefore, a trend is to combine classical chips with quantum computing chips.
For a large-scale practical problem, we need intelligent algorithms to decompose the problem and hand over the difficult parts to quantum chips to solve. Moreover, this difficult problem cannot be too large; if it is, it needs to be further broken down until the scale is small enough to be handled by NISQ quantum chips. Finally, the answers from these two systems are assembled to provide a final solution.
In the future, it is believed that this classical plus quantum hybrid computing approach can definitely be used to solve practical artificial intelligence problems.
Furthermore, as the computing power of artificial intelligence continues to increase, there may be an important research direction where artificial intelligence is used for chip design, in other words, using chips to develop better chips, essentially chip self-evolution.
At the 2020 ISSCC conference, the Google team revealed that they are using their TPU chips in integrated circuit design, such as in the circuit layout phase. The latest result is that AI completed the work in just 6 hours that previously took several weeks, and it did it better, reducing the number of wiring and improving area utilization.
In the future, artificial intelligence EDA software based on classical chips, or artificial intelligence EDA based on classical plus quantum hybrid computing, will gradually mature, bringing unprecedented advancement to chip technology.
4. Cutting-Edge Technology IV: Neuromorphic Chips
On July 15, 2020, the UK-based company Graphcore unveiled an AI chip manufactured using TSMC's 7-nanometer process, integrating a staggering 59.4 billion transistors. This is a massive number, considering the human brain has around 90 billion neurons.
Now, can intelligence be achieved on such a scale of nearly 600 billion? It's hard to say because the computing model of chips is significantly different from the human brain.
The current common computing model for chips is known as the Von Neumann architecture, where data storage and processing are separate. For instance, in our computers, data is stored in memory, and processing takes place in the CPU's processing unit. Each time a calculation is made, the CPU's control unit fetches the data from memory to the processing unit based on software commands.
However, this process is highly inefficient.
For example, a significant issue in computers today is the "memory wall," where the amount of data in memory keeps increasing, the CPU's processing speed accelerates, but the information channel between them remains narrow, like a dumbbell.
It's estimated that 99% of computing time and energy are spent on data transmission, with only 1% actually used for computation.
In contrast, the brain's computing model, known as "compute-in-memory," all takes place within neurons. Therefore, despite performing complex abstractions and reasoning, the brain consumes only 20 watts of power, far lower than current CPU chips.
There is a technology known as neuromorphic chips, aiming to mimic the brain's computing model. The key point of neuromorphic chips is the integration of computation and storage.
How can this be achieved? Several technologies can accomplish this, with the most promising example being memristors.
Memristors refer to devices whose resistance changes based on the current passing through them. In other words, the previous computation result is stored in the resistance, influencing the next calculation.
This mechanism mirrors the working principle of neurons in our brain. By utilizing this new device structure and computing model, neuromorphic chips hold great promise for high-performance, low-power intelligent computing. It's even speculated that once reaching a certain scale, new intelligent phenomena may emerge.
Conclusion
The evolution of chips has been marked by twists and breakthroughs, from early vacuum tubes to transistors, then MOSFETs, and onto large-scale integrated circuits. Each advancement has propelled the performance and functionality of electronic devices.
The continuous emergence of new materials, processes, and technologies signals immense potential and room for development in chip technology. This implies that we can anticipate smaller, more powerful chips, bringing forth more innovations in areas like smartphones, IoT, and artificial intelligence.
This ongoing progress epitomizes the evolution of technology, creating endless possibilities in our digital era. From quantum physics to the present, the development of chip technology has not only profoundly changed our way of life but also showcased the determination and immense power of human innovation.